Original research | Peer reviewed |
Cite as: van Riet MMJ, Bos EJ, Ampe B, et al. Long-term impact of zinc supplementation in sows: Impact on zinc status biomarkers and performance. J Swine Health Prod. 2018;26(2):79–94.
Also available as a PDF.
SummaryObjectives: To evaluate the long-term impact of zinc (Zn) supplementation on performance and Zn status biomarkers in sows and on whether this possible impact depends on housing conditions. Materials and methods: Six groups of sows were allotted to group housing on two different floor types during gestation. Within each group, sows were randomly allocated to one of three diets varying in the amount of Zn supplemented (0, 50, or 100 mg added Zn per kg diet; 50% ZnO:50% organic Zn) to a basal diet containing 46.6 and 128.9 mg Zn per kg during gestation and lactation, respectively. Blood was collected at days 0, 50, 108, and 143 of every cycle and analyzed for plasma Zn and copper and serum metallothionein (MT) concentrations. After slaughter, mineral concentrations of metacarpals, liver, and abaxial horn wall were determined. Results: Dietary Zn supplementation beyond basal dietary Zn concentrations did not influence serum MT concentrations (P = .77) and Zn concentrations in blood plasma (P = .13), liver (P = .54), bone (P = .26), and horn wall (P = .39). The 100-mg Zn per kg supplemented sows had lower bodyweight, body condition score, and backfat thickness (P < .001). The lack of impact of Zn supplementation may have been (partly) attributed to the unexpected high supply of Zn through premix in the lactation diet. Implications: Under these study conditions, commercially grown sows might not need Zn supplementation during gestation when their basal diet contains Zn with phytase. | ResumenObjetivos: Evaluar el impacto a largo plazo del suplemento de zinc (Zn) en el desempeño y en los biomarcadores de nivel de zinc en hembras, y si este posible impacto depende de las condiciones del alojamiento. Materiales y métodos: Durante la gestación, se asignaron seis grupos de hembras en alojamiento grupal en dos diferentes tipos de piso. Dentro de cada grupo, las hembras fueron asignadas aleatoriamente a una de tres dietas variando en la cantidad de Zn suplementado (0, 50, ó 100 mg de Zn adicionado por kg de dieta; 50% OZn:50% Zn orgánico) a una dieta básica con un contenido de 46.6 y 128.9 mg de Zn por kg, respectivamente durante la gestación y lactancia. Se tomaron muestras de sangre en el día 0, 50, 108, y 143 de cada ciclo y se analizaron en busca de concentraciones de Zn, cobre, y metalotioneína (MT por sus siglas en inglés) en suero. Después del sacrificio, se determinaron las concentraciones de minerales de los metacarpos, hígado, y pared abacial de la pezuña. Resultados: El suplemento dietético de Zn extra a las concentraciones dietéticas básicas no afectó las concentraciones de suero de MT (P = .77), ni las concentraciones de Zn en el plasma de la sangre (P = .13), hígado (P = .54), hueso (P = .26), y pared de la pezuña (P = .39). Las hembras suplementadas con100-mg de Zn por kg tuvieron un peso corporal, puntaje de condición corporal, y grosor de grasa de lomo (P < .001) menor. La falta del impacto de la suplementación de Zn, puede atribuirse (parcialmente), a la inesperada a alta inclusión de Zn en la premezcla de la dieta de lactancia. Implicaciones: Bajo las condiciones de este estudio, las hembras criadas comercialmente pueden no necesitar un suplemento de Zn durante la gestación cuando su dieta básica contenga Zn con fitasa. | ResuméObjectifs: Évaluer l’impact à long terme d’une supplémentation en zinc (Zn) sur les performances et les biomarqueurs du statut du Zn chez les truies et si cet impact possible dépend des conditions d’hébergement. Matériels et méthodes: Six groupes de truies ont été répartis en hébergement en groupe sur deux types différents de plancher durant la gestation. À l’intérieur de chaque groupe, les truies ont été aléatoirement assignées à une des trois diètes qui variaient quant à la quantité de Zn ajoutée (0, 50, ou 100 mg de Zn ajoutés par kg de nourriture; 50% ZnO:50% Zn organique) à l’aliment de base contenant 46,6 et 128,9 mg de Zn durant la gestation et la lactation, respectivement. Du sang a été prélevé aux jours 0, 50, 108, et 143 de chaque cycle et analysé pour les concentrations de Zn et de cuivre plasmatiques et pour les concentrations de métallothionéine (MT) sériques. Suite à l’abattage, les concentrations minérales ont été déterminées dans les métacarpes, le foie, et la paroi abaxiale de l’onglon. Résultats: Un supplément de Zn alimentaire au-delà des concentrations alimentaires de base du Zn n’a pas influencé les concentrations sériques de MT (P = 0,77) et les concentrations de Zn dans le plasma (P = 0,13), le foie (P = 0,54), l’os (P = 0,26), et la paroi de l’onglon (P = 0,39). Les truies ayant reçu le supplément de 100 mg par kg avaient des valeurs inférieures pour le poids corporel, le pointage de condition corporelle, et l’épaisseur du gras dorsal (P < 0,001). Le manque d’impact du supplément de Zn pourrait (en partie) être attribué à une quantité élevée inattendue de Zn via un pré-mélange dans la diète de lactation. Implications: Dans les conditions de la présente étude, les truies adultes ne nécessiteraient pas de supplémentation en Zn durant la gestation lorsque leur diète de base contient du Zn avec de la phytase.
|
Keywords: swine, dietary zinc concentration, rubber top layer flooring, zinc status biomarkers, performance
Search the AASV web site
for pages with similar keywords.
Received: December 23, 2016
Accepted: July 11, 2017
Zinc (Zn) metabolism changes throughout the reproductive cycle of sows to support fetal growth and development and milk production, as found in women.1-3 The underlying physiological processes seem to regulate these adaptations, but other factors, such as dietary Zn concentration, may affect the capacity to adapt. Across species, insufficient dietary Zn intake during gestation and lactation may result in reproductive failure.4-6
In sows, dietary Zn intake may change concentrations of Zn status biomarkers, such as plasma Zn and body-tissue Zn concentrations.7-10 In weaned piglets, plasma Zn concentration was affected by dietary Zn level.11 However, another study in sows showed increased plasma Zn concentrations only when Zn was added to the diet at levels above 500 mg Zn per kg.12,13
Most of the studies in sows that observed changes in plasma Zn concentrations are dated (published between 1967 and 1996), whereas the reproductive capacity of sows increased over time, and these studies used a small number of sows.14 None of these studies evaluated the responses of the cysteine-rich protein metallothionein (MT), which is important for absorption and storage of Zn. In addition, these studies each tested only one Zn supplementation level (between 42 and 5000 mg added Zn per kg) against a non-supplemented control group with a dietary Zn concentration between 10 ([semi]purified) and 35 mg Zn per kg to induce Zn deficiency. Furthermore, these studies did not evaluate the pattern of (changing) concentrations throughout gestation and lactation over multiple reproductive cycles. It is therefore unknown whether differences among dietary treatment groups are dependent on the reproductive phase and (or) cycle.
Therefore, the objective of the present study was to evaluate the impact of dietary Zn concentration on the profile (concentration and fluctuation) of Zn status biomarkers and performance characteristics in sows over three reproductive cycles. The chosen dietary range covered the array between low (marginal) and maximum allowed dietary Zn concentration in the European Union (maximum 150 mg Zn per kg). This longitudinal study of dietary Zn supplementation was performed on two different floor types during gestation, allowing a broader conclusion across housing conditions.
Materials and methods
All experimental procedures involving these animals were approved by the Institute for Agricultural and Fisheries Research’s Ethics Committee for animal experiments.
This longitudinal 2 × 3 factorial experiment was conducted according to the institutional and national guidelines for the care and use of animals.
Animals, housing, and management
Six groups of non-lame primiparous sows (total n = 131 gilts; RA-SE Genetics) were sequentially enrolled in the study when their locomotion score was ≤ 60 mm on a 150-mm tagged visual analogue scale (tVAS). First, during a quarantine period of 4 to 6 weeks, these purchased gilts were group-housed on concrete floors with straw bedding. The experimental period started 10 days before the first insemination (233 ± 12 days old at insemination). The six successive groups (n = 21 ± 4 sows per group; 3-week interval between groups) were monitored during three reproductive cycles. Body weight, backfat thickness, and body condition score (BCS, scale from 0 to 5) at the start of the study were 149 ± 21 kg, 16 ± 4 mm, and 3.0 ± 0.5, respectively (mean ± standard deviation [SD]) and did not differ between treatment groups (data not shown). A sow that had to be removed (n = 36) before the end of the experiment was replaced by a new gilt.
During the experimental period, sows were housed in individual gestation crates 10 days before their first insemination (day -10, start of the study; insemination at day 0) and from weaning (day -7) until 4 weeks after insemination (day 28) in their successive reproductive cycles. During mid- and end of gestation (day 28 to day 108), the sows were housed in static production groups. An automated feeding system was used with individual sow recognition through an electronic transponder in the sow’s ear. The group-housing facility consisted of four pens (4.45 m × 18.70 m); more detailed information on housing conditions is described in Bos et al.15 Two of the four pens, oriented diagonally to each other, had a similar floor type: either concrete slats and solid concrete lying areas or concrete slats covered with a rubber top layer (EasyFix; Rubber Products Ltd, Galway, Ireland) and rubber lying mats (Gummiwerk Kraiburg Elastik GmbH & Co Kg, Tittmoning, Germany) on 50% of the solid concrete floor area (“concrete” or “rubber floor” type, respectively). Each group was alternately assigned to the concrete or rubber floor type during group housing for the entire experiment. Per pen, two rubber balls and a static and dynamic rotating grooming brush were provided as environmental enrichment. The sows were housed individually in farrowing crates from 1 week before expected parturition until weaning (day 108 to day 143).
After weaning of the third reproductive cycle (end of the study), sows that had participated for at least 12 months (ie, sows that had completed at least two cycles, n = 95) in the experiment were slaughtered in a commercial slaughterhouse. This threshold was chosen on the basis of literature on dairy cows, postulating that the effect of dietary Zn on claw quality is dependent on study duration.16-18 The impact of dietary Zn on claw quality is presented in a previous publication.19 The sows were transported to the abattoir on the afternoon of the day before slaughter. Both front claws were marked with a coloured tie wrap to distinguish between left and right front claws. The next morning, the sows were slaughtered and both front claws were removed at the carpal joint before they entered the scalding vat to preserve the metacarpal bones and claw structures, and the whole liver was collected. The claw structures for examination, the liver, and the remaining part of the front claws, including metacarpal bones, were subsequently frozen at -20°C.
Dietary treatment
All purchased primiparous sows (gilts) were fed ad libitum during the quarantine period. The pre-experimental gestation diet was formulated according to National Research Council (NRC) recommendations20 and commercial standards for gestating sows, containing 895 g per kg dry matter (DM), 127 g per kg crude protein, 302 g per kg neutral detergent fibre (NDF), 155 g per kg acid detergent fibre (ADF), 24 g per kg acid detergent lignin (ADL), 28 g per kg crude fat, 70 g per kg crude ash, 129 g per kg starch, 66 g per kg sugar, 121 mg per kg Zn (originating from ingredients and from 100 mg Zn per kg added as ZnO via premix), and 4.7 g apparent ileal digestible lysine per kg diet.
Throughout the experimental period, sows were fed a gestation and lactation diet formulated according to NRC recommendations20 and commercial standards (Table 1 and Table 2) except for Zn. Phytase was added via the premix to simulate practical conditions. The gestation diet was offered 7 days before the first insemination or after weaning of the preceding reproductive cycle (day -7) until 1 week before parturition (day 108). The sows were fed twice daily from day -7 to the first 4 weeks of gestation (day 28), in total 2.3 kg per day, whereas during mid- and end of gestation (day 28 to day 108), sows were fed 2.6 kg per day. The lactation diet was provided from 1 week before parturition until weaning (day 108 to day 143). The sows were fed twice daily and received 3 kg feed provided in two equal portions the week before parturition (day 108 to day 115). After parturition, per suckling piglet, 0.25 kg of feed was gradually supplied in addition to 3 kg feed (eg, a sow with 12 piglets received 6 kg of feed per day), also provided in two equal portions per day.
Table 1: Ingredient composition of the gestation and lactation diets
Ingredients (g/kg as fed) | Gestation | Lactation |
---|---|---|
Wheat | 180 | 213 |
Barley | 180 | 100 |
Maize | 152 | 250 |
Wheat middling | 150 | 23 |
Beet pulp | 120 | 43 |
Soybean meal | 89 | 166 |
Soybeans heated | NA | 12 |
Soybean oil | 21 | NA |
Alfalfa meal | 47 | 94 |
Beet molasses | 30 | 30 |
Premix 3%* | 30 | NA |
Premix 2.75%† | NA | 27.5 |
Lard | NA | 30 |
Limestone | NA | 9.4 |
L-Valine | NA | 0.9 |
L-Threonine | 0.8 | 0.7 |
DL-Methionine | 0.7 | 0.3 |
L-Lysine HCL | 0.1 | 0.5 |
L-Tryptophan | NA | 0.1 |
Salt | 0.05 | NA |
* Premix 3% included per kg total gestation diet (analyzed Zn concentration in premix is 260 mg/kg) are presented in Supplementary materials.19
† Premix 2.75% included per kg total lactation diet (analyzed Zn concentration in premix is 4366 mg /kg) are presented in Supplementary materials.19
NA = not applicable (ingredients not added to the gestation or lactation diet).
Table 2: Analyzed and calculated* nutrient composition of the gestation and lactation diets†
Chemical analysis (g/kg) | Gestation | Lactation | ||||
---|---|---|---|---|---|---|
0 | 50 | 100 | 0 | 50 | 100 | |
DM | 877.4 | 876.9 | 877.1 | 880.0 | 878.3 | 879.6 |
Crude ash | 56.9 | 56.9 | 56.7 | 62.8 | 63.0 | 63.0 |
Crude protein | 136.7 | 136.9 | 136.8 | 160.8 | 161.0 | 160.7 |
Crude fat | 41.2 | 41.7 | 41.6 | 51.6 | 52.0 | 51.3 |
Crude fiber | 64.5 | 65.0 | 66.3 | 58.1 | 61.1 | 58.7 |
Starch | 277 | 270 | 268 | 313 | 304 | 314 |
Sugar | 55.8 | 56.2 | 55.2 | 55.4 | 55.2 | 53.6 |
Acid detergent fiber | 72.0 | 72.4 | 68.5 | 54.8 | 54.1 | 60.3 |
Neutral detergent fiber | 167 | 162 | 159 | 121 | 118 | 116 |
Acid detergent lignin | 9.9 | 10.6 | 11.5 | 6.8 | 6.3 | 7.0 |
Ca | 8.1 | 8.6 | 9.1 | 12.3 | 12.1 | 10.8 |
P | 4.4 | 4.3 | 4.3 | 5.0 | 4.9 | 5.0 |
Cu (mg/kg)‡ | 18.6 | 14.1 | 13.8 | 20.9 | 20.8 | 19.9 |
(15-25) | (13-15) | (13-15) | (19-22) | (19-22) | (19-22) | |
Zn (mg/kg)‡ | 46.6 | 81.9 | 124.4 | 128.9 | 184.3 | 229.0 |
(45-49) | (77-91) | (119-132) | (116-137) | (167-209) | (206-256) | |
ID Lysine | 6.0 | 7.9 | ||||
ID Methionine | 2.3 | 2.9 | ||||
ID Methionine + cysteine | 4.0 | 4.2 | ||||
ID Threonine | 4.3 | 5.5 | ||||
ID Tryptophan | 1.2 | 1.6 | ||||
ID Arginine | 6.6 | 8.3 | ||||
ID Leucine | 7.6 | 10.1 | ||||
ID Isoleucine | 3.8 | 5.1 | ||||
ID Histidine | 2.7 | 3.3 | ||||
ID Valine | 4.5 | 6.6 | ||||
ID Phenylalanine | 4.7 | 6.2 | ||||
NEv (MJ/kg)§ | 9.0 | 9.4 |
* Apparent ID amino acids and NEv for pigs were calculated according to the feed tables of the Centraal Veevoederbureau (CVB, the Netherlands), 2007.
† Dietary treatment is presented as 0-, 50-, or 100-mg Zn/kg supplemented to the basal diet containing 46.6 mg Zn/kg and 128.9 mg Zn/kg during gestation and lactation, respectively.
‡ Zn and Cu concentration are the average value of multiple feed sample analyses. Ranges for concentrations of both minerals in the gestation and lactation diets over time are presented between parentheses. The analyzed Zn concentration of the premix in the gestation diet was 260 mg/kg, which represent 7.8 mg Zn/kg in the final diet with 3% premix. The analyzed Zn concentration of the premix in the lactation diet was 4366 mg/kg, which represents 120 mg Zn/kg in the final diet with 2.75% premix.
§ Net energy for pigs is expressed as MegaJoules (MJ) per kg.
DM = dry matter; Ca = calcium; P = phosphorus; Cu = copper; Zn = zinc; ID = ileal digestible; NEv = net energy.
Throughout the experiment, all sows had ad libitum access to drinking water, except in the first 4 weeks of gestation, during which water was automatically provided through nipple drinkers for 15 minutes every hour and for 45 minutes while feeding in order to reduce water spillage.
Within each of the six static production groups, equal numbers of sows were randomly allocated to three dietary treatment groups, depending on the number of sows. The dietary treatments differed in Zn concentration: Zn not supplemented; Zn originated from ingredients only, 50 mg Zn per kg supplemented; and 100 mg Zn per kg supplemented. The Zn supplement comprised 50% inorganic Zn as ZnO (75% Zn) (33.3 or 66.6 g ZnO per 1000 kg feed, INVE Belgium N.V., Baasrode, Belgium), and 50% organic Zn as Availa Zn containing 10% Zn in an amino acid complex: single amino acids from hydrolysed soy protein (molar ratio 1:1, 250, or 500 g Availa Zn per 1000 kg feed, Zinpro Corporation, Eden Prairie, Minnesota).
Sample collection and measurements
Performance characteristics (body weight [BW], backfat thickness, body condition score [BCS], and reproductive performance) and Zn status biomarkers (liver and bone mineral concentration) were determined in all sows. For plasma Zn and copper (Cu) concentration, serum MT concentration and horn wall Zn concentration, 36 sows (12 of each dietary treatment group and at least one of each static production group) were selected. These sows were selected according to three criteria: three reproductive cycles completed, remained in their group of origin (ie, the group the sow was allocated to at the start of the experiment; repeat breeders that were transferred to another group receiving the same feed treatment and floor type, were not selected); and housed in their group during the entire gestation period (eg, sow was not separated from the group during group-housing period).
Performance characteristics
Body weight, backfat thickness, and BCS were determined to monitor sows’ health at day -10 (baseline, start of the study) and then on day 0 (insemination), day 20 (only backfat thickness and BCS), and (or) day 28 (only BW and BCS), day 108, and day 140 to day 143 (around weaning) of every reproductive cycle. Backfat thickness was determined between the 3rd and 4th last ribs, 7 cm from the left and right side of the vertebrae (P2 position). After lubricant was applied to P2, backfat measurements were determined, alternating between the left and right sides (Renco Lean Meater-12 60566; Renco Corporation, Minneapolis, Minnesota). If the difference between left and right was 2 mm or more, the measurements were repeated up to three times. The average thickness was used for further calculations. Body condition score was determined according to Evans21 using an ordinal scale including five categories from score 1 (emaciated) to score 5 (overly fat). A BCS of three represents the ideal condition.21
Reproduction performances (number of piglets born alive, average bodyweight [kg] of piglets born alive, number of stillborn piglets, number of weaned piglets, and average bodyweight [kg] of weaned piglets) were recorded. Cross-fostering between dietary treatments and provision of creep feed (transitional feed) to the piglets from day 10 postpartum were standard practice within the farm.
Zinc status biomarkers
Zinc status biomarkers were determined after blood collection at day 0 (insemination), day 50, day 108, and day 143 (weaning) every reproductive cycle, and tissue collection after slaughter.
Blood samples (20 mL) were taken from all sows within a group before feeding in the morning (between 8:30 am and 9:00 am) after overnight fasting of at least 18 hours. Blood samples were collected from the jugular vein using stainless steel needles and plastic syringes, and deposited in one heparin and one serum vacuum tube (Terumo Europe, Leuven, Belgium). One mL of heparinized blood was used to determine hematocrit (centrifuged 2749g, 30 minutes, 20°C) partly to monitor the sows’ health. The remainder was centrifuged (1500g, 10 minutes, 4°C) and plasma was divided between two 5-mL disposable polystyrene tubes. The tubes were stored for 24 hours at -20°C and then transferred to storage at -80°C until analysis of plasma Zn and Cu concentration. The vacuum serum tubes were centrifuged (1500g, 10 minutes, 4°C) after standing overnight at 4°C to allow clotting. Serum samples were divided between two 5-mL disposable polystyrene tubes, stored for 24 hours at -20°C and then at -80°C until analysis of serum MT concentration.
Plasma samples were deproteinized (Randox ZN2607; Randox Laboratories Ltd, Crumlin, United Kingdom) and the remaining supernatant was used within 2 hours to determine plasma Zn or Cu concentrations as described in Van Riet et al.22 Plasma Zn concentration was determined spectrophotometrically (EZ reader 400; Biochrom Ltd, Cambridge, United Kingdom) using a commercial colorimetric diagnostic kit (Randox kit, ZN2341; Randox Laboratories Ltd, Crumlin, United Kingdom). The observed plasma Zn concentration was interpolated from the multipoint standard calibration curve. The inter- and intra-assay CVs were 1.3% and 1.1%, respectively. The minimum and maximum recovery was 99.5% and 119.8%, respectively.22 Plasma Cu concentrations were determined spectrophotometrically (Ultrospec IIE; LKB Biochrom Ltd) using a commercial colorimetric diagnostic kit (Randox kit, CU2340, Randox Laboratories Ltd). The plasma Cu concentration was interpolated from the multipoint standard calibration curve. The inter- and intra-assay CVs were 1.8% and 1.1%, respectively. The minimum and maximum recoveries were 96.4% and 103.7%, respectively.22 Serum MT concentration was determined spectrophotometrically (EZ reader 400; Biochrom Ltd) using competitive ELISA (Porcine Metallothionein [MET] ELISA kit, E07M0030; BlueGene Biotech Co, Shanghai, China). Serum MT concentration was interpolated from the multipoint standard calibration curve. Serum MT concentrations below the detection limit (0.1 ng per mL) were corrected using the equation: detection limit ÷ √2. The inter- and intra-assay CVs were 3.3% and 2.0%, respectively. The certificate of analysis reported a recovery between 94% and 103%. For quality control, serum samples were spiked with 5 ng per mL and 10 ng per mL MT. The recoveries of spiked MT were 96.6% and 94.3%, respectively.
Body tissues (liver, bone, horn wall) were collected after slaughter for mineral analyses. Livers were cooled during transport. The left lateral and medial lobe (not including the right lobe with gall bladder) were sliced and ground using a mincer with 4.5 mm sieve (Kenwood kMix stand mixer with food grinder; Kenwood Ltd, Woking, United Kingdom). Then a representative homogenized sample (mean ± SD; 189 ± 23 g) was collected in a petri dish and stored at -20°C until lyophilisation. After lyophilisation, liver samples were oven dried at 103°C to a constant weight, and Zn and Cu concentrations were analyzed.
An abaxial horn wall sample of the lateral and medial digits of the right front claw was collected using an oscillating saw and the underlining tissues were removed. The remaining right front claw was stored at -20°C for collection of metacarpals. The horn wall samples were weighed and individually stored under vacuum at -20°C until testing for mechanical characteristics; procedures described in a previous publication.19 Post testing, the lateral and medial abaxial horn wall samples of 36 sows (dimensions: two samples of 20 mm length and 6 mm width with a variable thickness) were stored under vacuum at -20°C until analysis. Abaxial horn wall samples were dried at 103°C to a constant weight and analysed for Zn and Cu content.
The frozen right front claws with tie wrap were placed in a beaker (500 mL) filled with warm water with the claws pointing upwards to prevent charring of the metacarpal bones. The beaker was then placed in a warm water bath (75°C). After 24 hours, metacarpals 3 and 4 were collected and surrounding tissue removed. The metacarpals were weighed, oven dried for 16.45 hours at 65°C (Drying oven Binder APT line series ED; Binder GmbH Tuttlingen, Germany), and weighed again (Sartorius CP 324S, Göttingen, Germany). The lengths of metacarpals 3 and 4 (standardised as the length of each bone at the interior side located between metacarpals 3 and 4) was determined using a digital calliper. Metacarpals 3 and 4 were crushed in a vise lined with paper towels and stored at -20°C until fat extraction. The crushed metacarpals were defatted by extraction with petroleum ether (boiling point 40° to 60°C, ISO 6492A), dried at 103°C to a constant weight, and ashed at 825°C to a constant weight according to Bikker et al.23,24 The ash content of the fat-free dry matter was calculated on the basis of the weight of the bones before and after ashing. Metacarpals 3 and 4 were then ground according to a validated protocol (data not shown) that does not affect Zn and Cu concentrations using a ball mill (Retsch PM100; Led Techno NV; Heusden-Zolder, Belgium) with a 125-mL stainless steel grinding jar, seven stainless steel balls, and five droplets of ethanol (reagent grade) to obtain a homogenized sample. Grinding jar and balls were cleaned with sequentially distilled water (Type II), methanol (reagent grade), and distilled water (Type II) to prevent contamination. Equal aliquots (10 g) of the ground metacarpal 3 and 4 samples were combined to obtain one sample per sow and were analysed for calcium, phosphorus, Zn, and Cu content.
The left front claws were thawed for 24 hours and surrounding tissues were removed using a surgical knife. The weight and dimensions (length, thickness, and width) of metacarpals 3 and 4 were measured according to Combs et al25 (Figure 1).
Figure 1: Length (A), thickness (B), and width (C) dimensions of metacarpals 3 and 4 of left front claw. Measurements determined according to Figure 1 of Combs et al.25
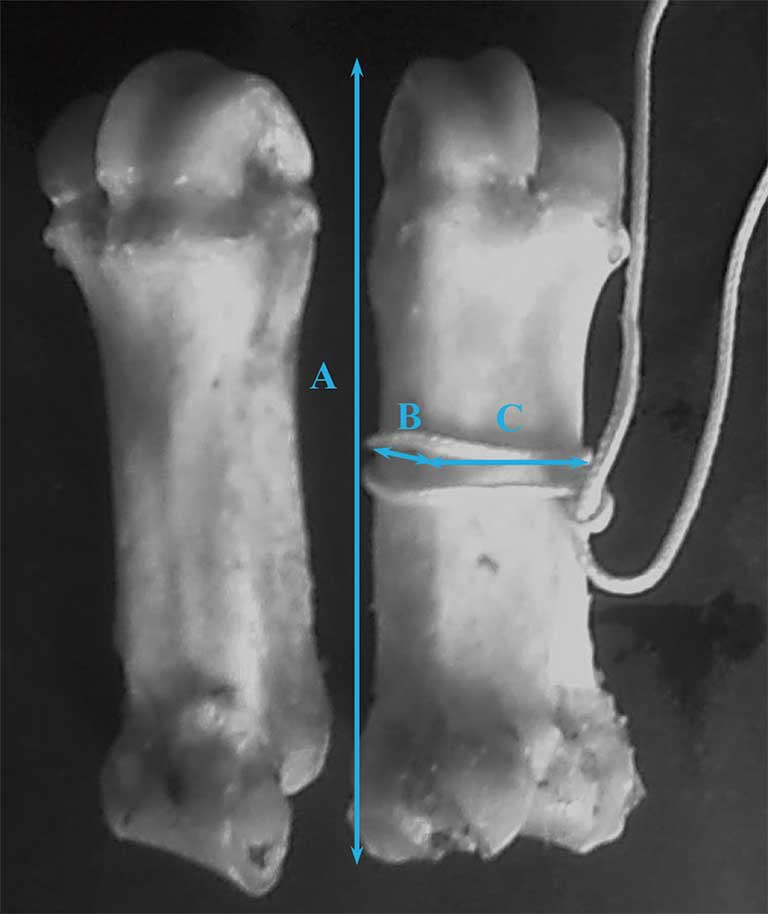
Chemical analysis
Feed samples of the gestation and lactation diets were collected from every batch and ground to pass through a 1-mm sieve for Near Infrared Spectroscopy evaluation and composited per dietary treatment group every 3 months for proximate analysis according to international standard methods accredited by ISO 17025.26 Dry matter, crude ash, crude protein, crude fat, calcium, and phosphorus content were determined according to 71/393/EEC, ISO 5984, ISO 5983-2, ISO 6492, ISO 6490/1, and ISO 6491, respectively. The American Oil Chemists’ Society (AOCS) approved procedure Ba 6a-05 was used to determine crude fiber content and the procedures described in Van Soest et al27 were used to determine ADF, NDF, and ADL.
The homogenized sample was further ground to pass a 0.5-mm sieve and three of five samples per dietary treatment were subjected to Zn and Cu analysis. Copper was analysed to assess possible antagonistic effects of Zn on Cu metabolism. Feed samples (1 g) were ashed and digested with HNO3 on a hot plate (150°C) for at least 30 minutes and transferred to a 50-mL flask. Liver samples (0.25 g) were diluted with HNO3:H2O2 for 12 hours and digested using microwave-assisted matrix digestion (MarsX; CEM, Matthews, North Carolina). Bone samples (1 g) were digested with 10 mL 6N HNO3 in a flask and diluted to 50 mL, and horn wall samples (approximately 0.8 g) were diluted in 10 mL 6N HNO3 for 12 hours, heated on a hot plate (150°C) for approximately 2 hours, and transferred to a 50-mL flask.
The Zn, Cu, and calcium concentration in the feed and tissue samples were determined by inductively coupled plasma optical emission spectrometry (ICP-OES; Vista MPX, Varian Inc Palo Alto, California), and bone phosphorus concentration was determined spectrophotometrically after matrix digestion.
Statistical analysis
Performance characteristics. Sow performance (BW, BCS, backfat thickness, reproduction) was analysed using a linear mixed model. Dietary Zn supplementation, floor type, phase within the reproductive cycle, parity, and all two-way interactions were included as fixed effects. Reproductive cycle, sow, and group were included in the models as random effects to correct for repeated measurements. A similar Poisson mixed model was used for the reproductive performance characteristics related to the number of born piglets (ie, number of piglets born alive, number of stillborn piglets, and number of weaned piglets).
Zn status biomarkers. Similar to the models for sow performance, linear mixed models were used to analyse the blood biomarkers and tissue characteristic data. Fixed and random effects included in the models differed according to time of sampling (eg, tissue collected at slaughter versus multiple blood collections throughout the reproductive cycle). For Zn status biomarkers in blood, dietary Zn supplementation, floor type, phase of the reproductive cycle, parity, and all two-way interactions were included as fixed effects, and reproductive cycle, sow, and group were included as random effects to correct for the repeated measurements. Observations for serum MT were transformed to a natural logarithm to obtain a normal distribution. For the mineral concentration in liver, bone, and horn wall and bone characteristic data, dietary Zn supplementation, floor type, parity, and all two-way interactions were included as fixed effects and sow and group were included as random effects.
Non-significant interactions and non-significant parity effects were excluded from all final models and P values of the main effects are presented. In case of a significant interaction, partitioned post-hoc P values are presented as tests of the simple effects of one variable for each level of the other variable. In the case of non-significant interactions, an all pairwise comparisons post-hoc test was performed. The P values of all post-hoc tests were corrected for multiple comparisons using the Tukey-Kramer method.
The analyzed data (except reproductive performance characteristics related to the number of born piglets) were considered to be sufficiently normally distributed, on the basis of the graphical evaluation (histogram and QQ-plot) of the residuals. All analyses were performed using SAS 9.4 (SAS Institute Inc, Cary, North Carolina).
Results
The interaction between dietary Zn supplementation and floor type was not significant for any of the outcome variables (P > .07) except for bone width dimension (P = .02). Therefore, main effects of dietary Zn supplementation and floor type, irrespective of the other main effects, are presented. Regarding the dietary Zn concentration, an unexpected high supply of Zn through premix in the non-supplemented lactation diet was analyzed that met NRC requirements during the last week of gestation until weaning (day 108 to day 143). However, the impact is unquantifiable.
Sows’ characteristics and farrowing performance
Throughout three reproductive cycles, 36 sows (27.5%), of which 9, 16, and 11 sows from the 0, 50, and 100 mg added Zn per kg treatment group, respectively, were removed from the experiment, and 21 of them were replaced with primiparous sows. Sows were removed for several reasons: spontaneous death (n = 10), euthanasia (rectal or uterine prolapse, severe locomotion disorders, n = 7), or reproductive failure after multiple attempts (n = 19). Seventy sows remained in their group of origin, whereas 26 sows were transferred to another group allocated to the same dietary treatment and floor type due to reproductive failure. In total, 92 of 95 sows that completed a minimum of two reproductive cycles were slaughtered at the end of the experiment. Of the other three sows, two died after their third parturition and the third sow was euthanized at the ILVO experimental farm immediately after the rest of the group was loaded for transport. The front claws and liver of this third sow were collected post mortem.
For the sows’ BW, an interaction between phase of reproductive cycle and dietary Zn supplementation was found irrespective of floor type. The mean BW of 100-mg Zn per kg supplemented sows was lower than that of the non-supplemented and 50-mg Zn per kg supplemented sows at day 28 (P = .001 and P < .001, respectively), day 108 (P < .001 and P < .001, respectively), and day 143 (P < .001 and P < .001, respectively), but not at the start of the study (Figure 2). No differences in sow BW were found between the non-supplemented and 50 mg added Zn per kg dietary treatment groups throughout the reproductive cycle (P = 1.0). Body condition score and backfat thickness were also lower for the 100 mg Zn per kg diet supplemented sows (Table 3). Body condition score remained constant between day 28 and day 50 (P = .13), increased towards day 108 (P < .001), and decreased towards day 143 (P < .001) of the reproductive cycle (Table 4). The BCS between day 28 and day 108 did not differ (P = .24) and BCS at day 143 was lower (P < .001) compared with other phases of the reproductive cycle. Backfat thickness increased from day 28 to day 108 (P = .008) and decreased towards day 143 (P < .001) of the reproductive cycle (Table 4). At day 108, backfat thickness was higher compared with other phases of the reproductive cycle (P < .001). A parity effect was found for BW (P = .005), BCS (P < .001), and backfat thickness (P < .001), showing higher BW and lower BCS and backfat thickness in the third parity (Table 4).
Figure 2: Fluctuations in body weight (kg) throughout three reproductive cycles in non-supplemented, 50-mg per kg, and 100-mg per kg supplemented sows (n = 36; 12 of each dietary treatment group). Values presented are means and their standard errors. Sows were group-housed between day 28 and day 108 of gestation on different floor types. The body weight of 100-mg Zn per kg supplemented sows was lower than those of the non-supplemented and 50-mg added Zn per kg supplemented sows at day 28, day 108, and day 143 (eg, interaction between phase of reproductive cycle and dietary Zn concentration, P < .001, linear mixed model). There were no differences between the non-supplemented and 50-mg added Zn per kg diet dietary treatment groups throughout the reproductive cycle (P = 1.0).
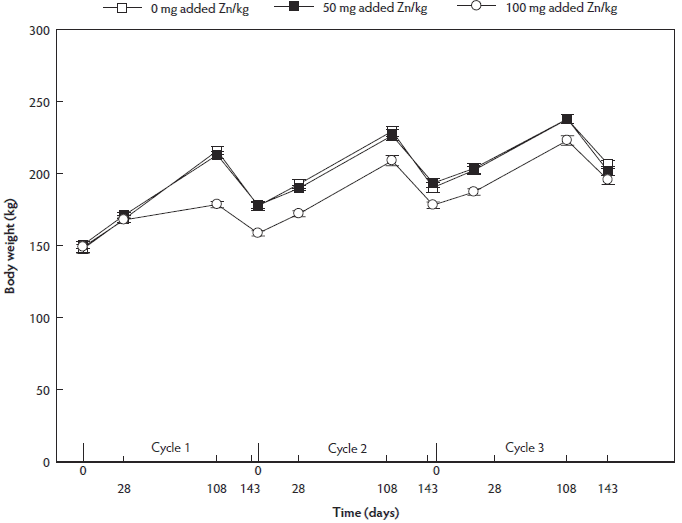
Table 3: Effect of dietary Zn supplementation on sows’ characteristics and farrowing performance when group housed on different floor types between day 28 and day 108 of gestation (n = 21 ± 4 sows per group)
Characteristic | Dietary treatment* × Floor type | SEM | P† | |||||
---|---|---|---|---|---|---|---|---|
0 × C | 50 × C | 100 × C | 0 × R | 50 × R | 100 × R | |||
BCS | 3.2 | 3.1 | 2.9 | 3.1 | 3.1 | 2.8 | 0.02 | < .001 |
Backfat (mm) | 14.8 | 14.0 | 12.7 | 14.8 | 15.0 | 12.5 | 0.20 | < .001 |
Hct (%) | 37.1 | 36.6 | 36.1 | 37.2 | 36.5 | 37.5 | 0.47 | .65 |
Farrowing performance | ||||||||
Piglets born alive (n) | 13.1 | 14.3 | 13.3 | 13.7 | 14.1 | 13.7 | 0.31 | .16 |
Ave BW, piglets born alive (kg) | 1.43 | 1.44 | 1.38 | 1.39 | 1.31 | 1.37 | 0.02 | .72 |
Stillborn piglets (n) | 1.1 | 1.1 | 1.3 | 0.8 | 1.0 | 0.7 | 0.15 | .77 |
Weaned piglets (n) | 10.8 | 11.7 | 10.7 | 11.2 | 11.0 | 11.0 | 0.28 | .45 |
Ave BW, weaned piglets (kg) | 7.45 | 7.63 | 7.06 | 7.48 | 7.46 | 7.15 | 0.10 | .01 |
* Dietary treatment is presented as 0, 50, or 100 mg Zn/kg supplemented to the basal diet containing 46.6 mg Zn/kg and 128.9 mg Zn/kg during gestation and lactation, respectively.
† As no interaction between phase of the reproductive cycle and dietary treatment for BCS, backfat thickness, or Hct was observed, the average BCS, backfat thickness, and Hct are presented. Level of significance is P < .05, linear mixed models, except for farrowing performance characteristics related to the number of born piglets for which a similar Poisson mixed model was used.
BCS = body condition score; C = concrete floor; R = rubber floor; Backfat = Backfat thickness; Hct = hematocrit; BW = body weight; Ave = average.
Table 4: Fluctuations in sows’ characteristics and farrowing performance throughout three reproductive cycles and over parity (n = 21 ± 4 sows per group)
Characteristic | Reproductive phase (days) | Parity 1 | Parity 2 | Parity 3 | SEM | P* | |
---|---|---|---|---|---|---|---|
Parity | Phase | ||||||
BCS | 28 | 3.4 | 3.1 | 2.9 | 0.10 | < .001 | < .001 |
108 | 3.2 | 3.3 | 3.2 | 0.07 | |||
143 | 2.7 | 2.7 | 2.9 | 0.03 | |||
Backfat (mm) | 20 | 16.1 | 11.9 | 11.1 | 0.30 | < .001 | < .001 |
108 | 17.7 | 16.2 | 16.0 | 0.40 | |||
143 | 12.4 | 11.0 | 11.5 | 0.33 | |||
Hct (%) | 0 | 40.9 | 37.9 | 37.5 | 0.70 | < .001 | < .001 |
50 | 40.8 | 37.9 | 36.2 | 1.23 | |||
108 | 37.4 | 36.1 | 35.5 | 0.63 | |||
143 | 34.5 | 33.6 | 33.4 | 0.73 | |||
Farrowing performance | |||||||
Piglets born alive (n) | 13.3 | 13.8 | 14.1 | 0.33 | .16 | NA | |
Ave BW of piglets born alive (kg) | 1.3 | 1.5 | 1.4 | 0.00 | < .001 | NA | |
Stillborn pigletss (n) | 1.1 | 0.6 | 1.2 | 0.17 | < .001 | NA | |
Weaned piglets (n) | 10.7 | 11.5 | 11.4 | 0.30 | .09 | NA | |
Ave BW of weaned piglets (kg) | 7.3 | 7.7 | 7.1 | 0.10 | < .001 | NA |
* P values are presented for parity and phase within the reproductive cycle. Level of significance is P < .05 using linear mixed models, except for farrowing performance characteristics related to the number of born piglets for which a similar Poisson mixed model was used.
BCS = body condition score; Backfat = Backfat thickness; Hct = hematocrit; BW = body weight; Ave = average; NA = not applicable.
Dietary treatment was not associated with number of piglets born alive, average BW of piglets born alive, number of stillborn piglets, or number of weaned piglets (Table 3). Piglets from sows that receieved 100-mg added Zn per kg diet had a lower BW at weaning than did those of non-supplemented and 50-mg added Zn per kg supplemented sows (Table 3). A parity effect was found for the number of stillborn piglets, which was lower in the second parity (P < .001), and for the average BW of piglets born alive, which was lower in the first parity (P < .001) (Table 4). The average BW of weaned piglets was lower in the third parity (P < .001) (Table 4).
Hematocrit (Hct; %) did not differ between dietary treatment groups (Table 3). The Hct remained constant between day 0 and day 50 (P = .91), decreased towards day 108 (P = .03), and then decreased until day 143 (P = .003) (Table 4). Hematocrit was lower in reproductive cycles 2 and 3 than in reproductive cycle 1 (P = .003 and P < .001, respectively) (Table 4).
Independent of dietary Zn supplementation, floor type had only minor influences on reproductive performance (P > .47 for number of piglets born alive, number of weaned piglets and average BW of weaned piglets; P = .095 for average BW of piglets born alive; and P = .044 for number of stillborn piglets).
Zn status biomarkers
Plasma Zn concentration did not differ between dietary treatment groups irrespective of floor type (P = .13). Generally, plasma Zn concentration decreased from insemination (day 0) to day 50 of gestation (P < .001), remained constant to day 108 of gestation (P = .999), and decreased until weaning (day 143) (P = .003). Plasma Zn concentration was lower in reproductive cycle 3 than in reproductive cycles 1 and 2 (P < .001 and P < .001, respectively) (Figure 3).
Figure 3: Fluctuations in plasma Zn concentrations (µg/dL) throughout three reproductive cycles in non-supplemented, 50-mg/kg and 100-mg/kg supplemented sows (n = 36, 12 of each dietary treatment group). Values presented are means and their standard errors. Sows were group-housed between day 28 and day 108 of gestation on different floor types. Plasma Zn concentration did not differ between dietary treatment groups (P = .13) or between floor types (P = .28). Plasma Zn concentration decreased from insemination (day 0) to day 50 of gestation, remained constant to day 108 of gestation, and decreased further towards weaning (day 143) (P < .001; linear mixed model). Plasma Zn concentration was lower in reproductive cycle 3 than in reproductive cycles 1 and 2 (P < .001).
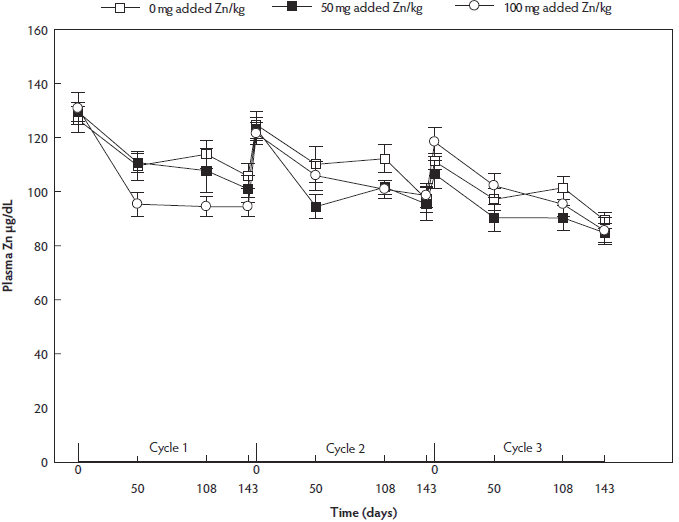
Plasma Cu concentration did not differ between dietary treatment groups (P = .37), but was lower at day 143 than at day 0 and day 50 (P = .005 and P = .03, respectively). Plasma Cu concentration was lower in reproductive cycle 3 than at reproductive cycles 1 and 2 (P = .04 and P = .008, respectively) (Figure 4).
Figure 4: Fluctuations in plasma Cu concentrations (µg/dL) throughout three reproductive cycles in non-supplemented, 50-mg per kg and 100-mg/kg supplemented sows (n = 36; 12 of each dietary treatment group). Values presented are means and their standard errors. Sows were group-housed between day 28 and day 108 of gestation on different floor types. Plasma Cu concentration did not differ between dietary treatment groups (P = . 37) or between floor types (P = .69), but was lower at day 143 than at day 0 or day 50 (P = .004, linear mixed model). Plasma Cu concentration was lower in reproductive cycle 3 than in reproductive cycles 1 and 2 (P = .006).
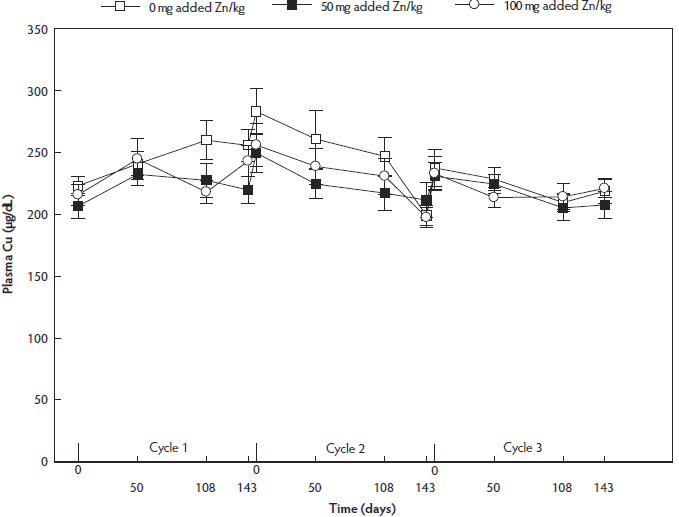
Serum MT concentration did not differ between dietary treatment groups (P = .77). It decreased from day 0 towards day 50 and day 108 and increased towards day 143 (P = .01, P < .001, and P = .04, respectively), but the MT concentration did not differ between day 50 and day 143 (P = .29). The serum MT concentration was lower in reproductive cycle 2 than in reproductive cycle 3 (P = .03) (Figure 5).
Figure 5: Fluctuations in serum metallothionein (MT) concentrations (ng/mL) throughout three reproductive cycles in non-supplemented, 50-mg/kg and 100-mg/kg supplemented sows (n = 36, 12 of each dietary treatment group). Values presented are means and their standard errors. Sows were group housed between day 28 and day 108 of gestation on different floor types. Serum MT concentration did not differ between dietary treatment groups (P = .77), but was lower for sows housed on the floor covered with a rubber top layer (P = .003). Serum MT concentration decreased from day 0 towards day 50 and day 108 and increased towards day 143 (P = .01, P < .001, and P = .04, respectively), but the MT concentration did not differ between day 50 and day 143 (P = .29; linear mixed model). Serum MT concentration was lower in reproductive cycle 2 than in reproductive cycle 3 (P = .03).
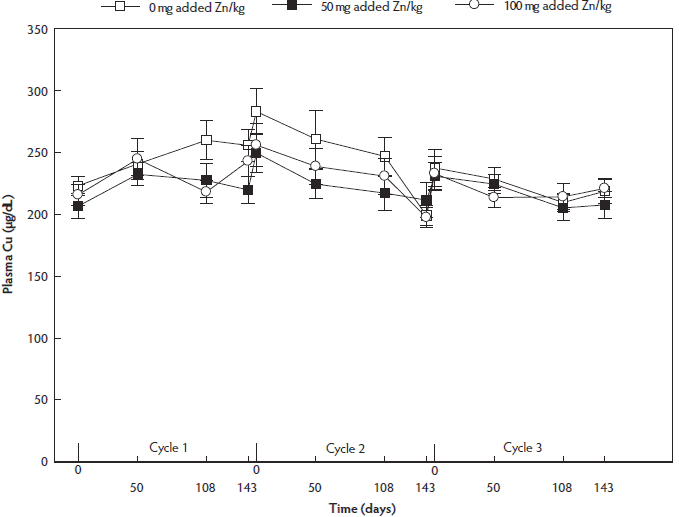
Floor type did not affect plasma Zn and Cu concentrations (P = .28 and P = .69, respectively). Serum MT concentration was lower for sows housed on rubber floors (P = .003) (Table 5).
Table 5: Effect of floor type on Zn status biomarkers (n = 21 ± 4 sows per group)
Zn status biomarker | Dietary treatment* × Floor type | SEM | P† | |||||
---|---|---|---|---|---|---|---|---|
0 × C | 50 × C | 100 × C | 0 × R | 50 × R | 100 × R | |||
Plasma Zn | 107.2 | 99.6 | 102.6 | 109.2 | 106.5 | 104.6 | 0.96 | .28 |
Plasma Cu | 238.2 | 234.3 | 221.6 | 237.7 | 207.1 | 231.4 | 2.22 | .69 |
Serum MT | 1.10 | 0.99 | 0.94 | 0.54 | 0.60 | 2.15 | 0.03 | .003 |
* Dietary treatment is presented as 0, 50, or 100 mg Zn/kg supplemented to the basal diet containing 46.6 mg Zn/kg and 128.9 mg Zn/kg during gestation and lactation, respectively.
† As no interaction between phase of the reproductive cycle and floor type for Zn status biomarkers was observed, the average values are presented. Level of significance is P < .05, linear mixed models.
Zn = zinc; Cu = copper; MT = metallothionein; C = concrete floor; R = rubber floor.
Liver weight, liver Zn and Cu concentrations, bone Zn, Cu, calcium, and phosphorus concentrations, and horn wall Zn and Cu concentrations did not differ between dietary treatment groups nor between floor type treatments (Table 6). An interaction for the bone width dimension was found between dietary Zn supplementation and floor type (Table 7). Other bone characteristics did not differ between dietary or floor type treatment groups (Table 7).
Table 6: Effect of dietary Zn supplementation on mineral concentration of body tissues from slaughtered sows group-housed on different floor types between day 28 and day 108 of gestation (n = 92 sows for liver and bone, n = 36 sows for horn wall)
Body tissues | Dietary treatment* × Floor type | SEM | P† | ||||||
---|---|---|---|---|---|---|---|---|---|
0 × C | 50 × C | 100 × C | 0 × R | 50 × R | 100 × R | Zn | F | ||
Liver‡ | |||||||||
Total liver weight (g) | 2790.0 | 2912.5 | 2738.0 | 2892.2 | 2736.9 | 2601.3 | 54.9 | .43 | .59 |
Zn in FM (mg/kg) | 56.2 | 57.6 | 53.7 | 53.4 | 51.4 | 51.3 | 1.7 | .72 | .30 |
Total liver Zn (mg) | 155.0 | 162.5 | 147.2 | 152.1 | 137.0 | 133.4 | 5.2 | .54 | .22 |
Cu in FM (mg/kg) | 26.5 | 30.6 | 36.1 | 43.0 | 47.9 | 30.0 | 3.6 | .93 | .22 |
Total liver Cu (mg) | 75.2 | 81.7 | 99.4 | 121.3 | 142.1 | 81.6 | 10.7 | .87 | .21 |
Metacarpals 3 and 4 | |||||||||
Ash (g/kg FFDM) | 625.0 | 620.0 | 618.0 | 631.0 | 621.0 | 624.0 | 0.2 | .28 | .25 |
Zn in ash (mg/kg) | 57.6 | 50.6 | 56.6 | 63.5 | 54.1 | 64.0 | 2.2 | .28 | .19 |
Cu in ash (mg/kg) | 9.9 | 9.1 | 8.5 | 8.8 | 8.1 | 10.1 | 0.3 | .49 | .80 |
Ca in ash (mg/kg) | 394.9 | 395.6 | 397.3 | 395.1 | 394.6 | 394.1 | 0.8 | .86 | .40 |
P in ash (mg/kg) | 179.5 | 181.8 | 180.4 | 181.0 | 182.7 | 179.4 | 0.5 | .15 | .64 |
Zn in FFDM (g/kg) | 36.2 | 31.4 | 35.0 | 40.2 | 33.7 | 40.0 | 1.4 | .26 | .18 |
Cu in FFDM (g/kg) | 6.2 | 5.6 | 5.3 | 5.6 | 5.0 | 6.3 | 0.2 | .40 | .90 |
Ca in FFDM, (g/kg) | 246.6 | 245.2 | 245.5 | 249.2 | 245.2 | 245.8 | 0.9 | .43 | .55 |
P in FFDM (g/kg) | 112.1 | 112.7 | 111.5 | 114.2 | 113.5 | 111.9 | 0.5 | .38 | .24 |
Horn wall Zn | |||||||||
Dry matter (g/kg) | 761.2 | 757.0 | 774.6 | 768.3 | 779.6 | 775.0 | 3.6 | .46 | .39 |
Zn in DM (mg/kg) | 126.6 | 118.6 | 124.6 | 129.2 | 126.4 | 116.7 | 2.3 | .39 | .86 |
Cu in DM (mg/kg) | 4.0 | 4.2 | 4.1 | 3.8 | 3.8 | 3.6 | 0.2 | .94 | 1 |
* Dietary treatment is presented as 0, 50, or 100 mg Zn/kg supplemented to the basal diet containing 46.6 mg Zn/kg and 128.9 mg Zn/kg during gestation and lactation, respectively
† No interactions between dietary Zn supplementation and floor type were observed (P > .30, except for metacarpal Cu concentrations [P > .07]). These non-significant interactions were excluded from the final models and P values are presented for the main effect of dietary Zn supplementation (Zn) and for the main effect of floor type.
‡ Total liver weight may deviate due to losses during liver collection in the slaughterhouse. Metabolic weight is the BW0.75 of sows before transport to slaughterhouse and did not differ between treatment groups (P = .11) and floor type (P = .24). Level of significance is P < .05, linear mixed models.
C = concrete floor; R = rubber floor; Zn = zinc; F = floor type; Ca = calcium; P = phosphorus; FFDM = fat free dry matter; FM = fresh matter; CU = copper; DM = dry matter.
Table 7: Effect of dietary Zn supplementation on metacarpal characteristics from slaughtered sows group-housed on different floor types between day 28 and day 108 of gestation (n = 54 for left front claw and n = 36 for right front claw).
Metacarpals* | Dietary treatment† × Floor type | SEM | P‡ | ||||||
---|---|---|---|---|---|---|---|---|---|
0 × C | 50 × C | 100 × C | 0 × R | 50 × R | 100 × R | Zn | F | ||
Left front claw | |||||||||
Length (mm) | 90.6 | 89.8 | 88.1 | 89.7 | 92.0 | 91.2 | 0.4 | .57 | .15 |
Thickness (mm)§ | 19.7 | 20.7 | 19.8 | 19.8 | 20.3 | 20.1 | 0.1 | .10 | .46 |
Width (mm)§ | 17.3 | 17.3 | 16.7 | 16.2 | 16.7 | 17.7 | 0.1 | ** | |
Weight (g) | 43.6 | 45.3 | 41.4 | 43.3 | 45.8 | 45.6 | 0.7 | .29 | .24 |
Right front claw | |||||||||
Length (mm) | 88.6 | 88.2 | 88.7 | 89.3 | 90.2 | 88.8 | 0.3 | .94 | .26 |
Weight (g)¶ | 37.2 | 38.1 | 37.4 | 38.6 | 38.9 | 38.5 | 0.3 | .88 | .21 |
* Average of metacarpals 3 and 4 for length, weight, and (or) thickness and width.
† Dietary treatment is presented as 0, 50-, or 100-mg Zn/kg supplemented to the basal diet containing 46.6 mg Zn/kg and 128.9 mg Zn/kg during gestation and lactation, respectively.
‡ No interactions between dietary Zn supplementation and floor type were observed (P = .23, excluding P = .07 for bone thickness), except for bone width dimension. Non-significant interactions were excluded from the final models and P values are presented for the main effect of dietary Zn supplementation (Zn) and for the main effect of floor type. Level of significance is P < .05, linear mixed models.
§ Thickness and width dimensions of average metacarpal 3 and 4 of left front claw (Figure 1) were determined according to Combs et al.25
¶ Average metacarpal 3 and 4 of the right front claw were weighed after 24 hours at 75°C.
** Interaction of dietary Zn supplementation and floor type for bone width dimension, post-hoc partitioned P values are P = .41 (Concrete × dietary Zn supplementation) and P = .02 (rubber × dietary Zn supplementation).
C = concrete floor; R = rubber floor; Zn = zinc; F = floor type.
Discussion
Despite the considerable range in dietary Zn concentration among the treatments, the serum MT concentrations and concentrations of Zn in blood plasma, liver, bone, and horn wall did not respond to increased dietary Zn inclusion levels beyond basal dietary Zn concentrations in the present study. The lack of impact of Zn supplementation may have been (partly) attributed to the unexpected high supply of Zn through premix in the non-supplemented lactation diet that met NRC requirements during the last week of gestation until weaning (day 108 to day 143). Although sows received Zn above NRC requirements during this period, these results still suggest that the tested dietary range did not disturb Zn homeostasis, including the non-supplemented sows during gestation, as compared to earlier studies in rats.28-31 Because Zn homeostasis is very important to ensure that all body processes are optimally regulated, Zn flow is tightly regulated. Adjustments in the processes of Zn absorption and excretion as shown in rats and weaned piglets,14,28,30,32-34 may have also occurred in the present study. Although we did not measure absorption, it can be assumed that a higher Zn intake did not result in a proportionally higher absorption and utilisation of Zn, for example, due to the down-regulation of the transport protein ZIP4 in the lumen.35 Simultaneously, the amount of Zn in feces might increase due to reduced absorption and increased excretion when the transport protein ZnT1 and MT are upregulated in the lumen.35 Because MT binds Zn within the enterocyte, Zn is excreted when the enterocyte is sloughed off.34,36
Most other studies on the effect of dietary Zn levels found differences between treatment groups in plasma Zn7-10 and liver and bone Zn concentration in sows7,12,13,37 and piglets.23,38-42 Most of these studies, especially the studies on sows, compared the responses between a non-supplemented and supplemented treatment group without addition of phytase, though diets were (semi) purified or used to induce Zn deficiency and do not represent practical conditions. The dietary Zn concentration of the control group was lower (< 35 mg Zn per kg) in these studies than in the concentration of the non-supplemented diet with phytase in the present study during gestation (47 mg Zn per kg). The lower dietary Zn concentration of the other studies suggests that the non-supplemented sows were not able to maintain homeostasis and that those dietary Zn concentrations were insufficient. Phytase was added in the present study to simulate practical conditions, but this may have increased Zn status more than Zn supplementation without phytase, as found in piglets.23,40-42 However, in sows, addition of phytase did not affect the digestibility of Zn during gestation, but it did during lactation.43 The long-term impact of the addition of phytase on Zn status is unknown in sows during reproduction. Nonetheless, these findings suggest that the basal dietary Zn concentrations with phytase were adequate for sows in the present study. Similar observations were found in rats, where lowered distribution between tissues, decreased fecal Zn excretion, and decreased concentration of Zn in some tissues (digestive organs, hair, plasma, and skeleton) at insufficient dietary Zn concentrations were found, whereas differences between tissue Zn concentrations were negligible between adequate and excessive dietary Zn intake.30 At adequate and excessive dietary Zn concentrations, whole-body Zn concentration in rats seems to be unaffected.28-31 This indicates that excessive Zn is not absorbed but excreted, or that Zn is redistributed between tissues and that this redistribution is enhanced with increasing dietary Zn concentrations to protect tissues.14,44,45 However, disturbed homeostasis does not necessarily indicate that a sow is (marginally) deficient, because despite the differences between treatment groups for plasma Zn concentrations in most other studies in sows, the concentrations reported in these studies are within the range of 50 to 150 μg per dL. It is possible that the remarkable fluctuations throughout the reproductive cycles, independent of dietary Zn concentration, had already overruled the effects of different dietary Zn intake. In the present study, plasma Zn and serum MT concentrations fluctuated during the reproductive cycle, which is in agreement with observations in other sow studies,9,10,13,22,46 in women,1 and in sheep.47
Liver and bone are major storage organs for Zn in pigs. Especially bone seems to accumulate Zn with increasing dietary Zn concentrations compared to other Zn status biomarkers, which plateau at lower inclusion levels.23,41 Possibly in sows, liver and bone will accumulate Zn only when dietary Zn intake is substantially beyond Zn requirements, with or without addition of phytase, in an attempt to maintain Zn homeostasis. This suggests that in our study, the absorbed fraction was still at a level that did not require Zn accumulation in storage tissues. At excessive dietary Zn concentrations, Hill et al12,13 found a linearly increased liver Zn concentration in sows fed diets reaching pharmacological dietary Zn concentrations (> 500 mg added Zn per kg diet). At these concentrations, Zn absorption may have changed from a carrier-mediated to a passive-transport pathway as found in piglets.35 As a response, Zn absorption and Zn (re)distribution between body tissues are disturbed, resulting in increased Zn concentration in the liver.12,13,35 The diet provided during lactation had a considerable Zn concentration (129 mg Zn per kg diet), which might have prevented a potential lack during gestation, meaning that, in general, the Zn concentration in the lactation diet needs to be considered in evaluating the impact of dietary Zn supplementation during gestation.
In the present study, sows’ BW, backfat thickness, and BCS and the average BW of their weaned piglets were negatively affected for the 100-mg Zn per kg supplemented sows. Hill et al12,13 observed a similar effect in sows, but only at much higher levels (5000 mg added Zn per kg diet), possibly because phytase was not included in their diets. Studies in cattle also found decreased performance characteristics in their supplementation group (Fagari-Nobijari et al,48 150 mg added Zn per kg DM; and Dermauw et al,49 540 mg added Zn per cow per day). It is not yet fully understood why greater Zn supplementation would reduce performance. The response seems to be mainly caused by events in the first reproductive cycle. Feed intake, Zn reserves, and Zn requirements cannot explain the lowered performance in the present study, and no antagonistic effects on Cu, calcium, or phosphorus were observed, which is in agreement with other studies in sows12,13 and weaned piglets.34 Furthermore, no noteworthy situations were reported throughout the experiment that could have influenced the observed results. Possibly, although not evaluated in the present study, protein expression in the liver and pancreas may be altered, which is related to cellular stress responses, transport, metabolism, and signal transduction, without influencing liver or pancreas Zn concentration or hepatic mRNA MT expression as found in piglets.50,51 Further research is required. The poorer performance of the piglets seems a logical consequence of poorer sow performance.
No differences in the concentration of Zn status biomarkers were observed between dietary treatment groups in the present study, suggesting that Zn homeostasis was maintained and that Zn requirements were met, even for the non-supplemented sows. Floor type did not affect the observed responses of Zn status biomarkers. The reproductive phase had a more important effect on the measured parameters for Zn status with fluctuations noted throughout the cycle. The negative effect of higher dietary Zn supplementations on body weight of sows and piglets as apparently observed in other species suggests the need for additional mechanistic studies.
Implications
- Under the conditions of this study, commercially grown sows might not need supplemental Zn in their gestation diet beyond basal dietary Zn concentrations with phytase. On the basis of the assessment of Zn status biomarkers, there are no indications that Zn homeostasis is disturbed.
• During gestation, basal dietary Zn concentrations with phytase seem adequate for sows to maintain Zn homeostasis, but there is a need for mechanistic studies to evaluate the negative effect of higher dietary Zn supplementation on body weight of sows and piglets.
- Fluctuations of Zn status biomarkers throughout the reproductive cycle need to be taken into account when assessing Zn status.
Acknowledgements
This study was supported by the Institute for Promotion of Innovation through Science and Technology in Flanders (IWT, grant number 090938), and co-funded by Orffa, VDV Beton, Boerenbond, AVEVE, INVE and Boehringer Ingelheim. The authors thank the technicians M. van Yperen and T. Martens, animal caretakers at the ILVO experimental farm, ILVO colleagues and students, J. Buist, J. M. Muijlaert, laboratory personnel of participated departments, and personnel of the slaughterhouse in Eeklo (Belgium) for their much appreciated assistance and support. Thanks also to M. Levenson for English-language editing.
Conflict of interest
None reported.
Disclaimer
Scientific manuscripts published in the Journal of Swine Health and Production are peer reviewed. However, information on medications, feed, and management techniques may be specific to the research or commercial situation presented in the manuscript. It is the responsibility of the reader to use information responsibly and in accordance with the rules and regulations governing research or the practice of veterinary medicine in their country or region.
References
1. Donangelo CM, Vargas Zapata CL, Woodhouse LR, Shames DM, Mukherjea R, King JC. Zinc absorption and kinetics during pregnancy and lactation in Brazilian women. Am J Clin Nutr. 2005;82:118–124.
2. Maia PA, Figueiredo RCB, Anastácio AS, Porto da Solveira CL, Donangelo CM. Zinc and copper metabolism in pregnancy and lactation of adolescent women. Nutrition. 2007;23:248–253.
3. Caulfield LE, Donangelo CM, Chen P, Junco J, Merialdi M, Zavaleta N. Red blood cell metallothionein as an indicator of zinc status during pregnancy. J Nutr. 2008;24:1081–1087.
4. Smith OB, Akinbamijo OO. Micronutrients and reproduction in farm animals. Anim Reprod Sci. 2000;60-61:549–560.
5. Ashworth CJ, Antipatis C. Micronutrient programming of development throughout gestation. Reproduction. 2001;122:527–535.
6. Vazquez-Armijo JF, Rojo R, Salem AZM, Lopez D, Tinoco JL, González A, Pescador N, Dominguez-Vara IA. Trace elements in sheep and goats reproduction: a review. Trop Subtrop Agroecosyst. 2011;14:1–13.
7. Hoekstra WG, Faltin EC, Lin CW, Roberts HF, Grummer RH. Zinc deficiency in reproducing gilts fed a diet high in calcium and its effects on tissue zinc and blood serum alkaline phosphatase. J Anim Sci. 1967;26:1348–1357.
8. Hedges JD, Kornegay ET, Thomas HR. Comparison of dietary zinc levels for reproducing sows and the effect of dietary zinc and calcium on the subsequent performance of their progeny. J Anim Sci. 1976;43:453–463.
9. Kalinowski J, Chavez ER. Effect of low dietary zinc during late gestation and early lactation on the sow and neonatal piglets. Can J Anim Sci. 1984;64:749–758.
10. Kalinowski J, Chavez ER. Low dietary zinc intake during pregnancy and lactation of gilts. 1. Effects on the dam. Can J Anim Sci. 1986;66:201–216.
11. van Riet MMJ, Janssens GPJ, Cornillie P, Van Den Broeck W, Nalon E, Ampe B, Tuyttens FAM, Maes D, Du Laing G, Millet S. Marginal dietary zinc concentration affects claw conformation measurements but not histological claw characteristics in weaned pigs. Vet J. 2016;209:98–107.
12. Hill GM, Miller ER, Stowe HD. Effect of dietary zinc levels on health and reproductivity of gilts and sows through two parities. J Anim Sci. 1983;57:114–122.
13. Hill GM, Miller ER. Effect of dietary zinc levels on the growth and development of the gilt. J Anim Sci. 1983;57:106–113.
14. Jongbloed AW, Bikker P, van den Top AM. Copper and zinc requirements of high-producing reproductive sows. Confidential Report 244. Wageningen UR Livestock Research, Lelystad, The Netherlands. 2010;5–10.
15. Bos E-J, van Riet MMJ, Maes D, Millet S, Ampe B, Janssens GPJ, Tuyttens FAM. Effect of rubber flooring on group-housed sows’ gait, claw and skin lesions. J Anim Sci. 2016;94:2086–2096.
16. Hedges VJ, Blowey R, Packington AJ, O’Callaghan CJ, Green LE. A longitudinal field trial of the effect of biotin on lameness in dairy cows. J Dairy Sci. 2001;84:1969–1975.
17. Griffiths LM, Loeffler SH, Socha MT, Tomlinson DJ, Johnson AB. Effects of supplementing complexed zinc, manganese, copper and cobalt on lactation and reproductive performance of intensively grazed lactating dairy cattle on the South Island of New Zealand. Anim Feed Sci Tech. 2007;137:69–83.
18. Lethbridge LA. Lameness of dairy cattle: factors affecting the mechanical properties, haemorrhage levels, growth and wear rates of bovine claw horn. PhD dissertation. Massey University, New Zealand. 2009.
19. van Riet MMJ, Bos EJ, Ampe B, Bikker P, Vanhauteghem D, Van Bockstaele F, Cornillie P, Van Den Broeck W, Du Laing G, Maes D, Tuyttens FAM, Janssens GPJ, Millet S. Long-term impact of zinc supplementation in sows: Impact on claw quality. J Swine Health Prod. 2018;26:10–24.
20. NRC. Nutrient Requirements of Swine: 11th rev ed. National Academy Press: Washington DC. 2012.
21. Evans DG. The interpretation and analysis of subjective body condition scores. Anim Prod. 1978;26:119–125.
22. van Riet MMJ, Millet S, Nalon E, Langendries KCM, Cools A, Ampe B, Du Laing G, Tuyttens FAM, Maes D, Janssens GPJ. Fluctuation of potential zinc status biomarkers throughout a reproductive cycle of primiparous and multiparous sows. Brit J Nutr. 2015;114:544–552.
23. Bikker P, Jongbloed AW, Verheijen R, Binnendijk G, Van Diepen JTM. Zinc requirements of weaned piglets. Confidential Report 274, Wageningen UR Livestock Research, Lelystad, The Netherlands. 2011;1–31.
24. Bikker P, Dekker RA, van Diepen JTM, van Krimpen MM, Jongbloed AW, Millet S. Phosphorus requirements and retention in growing finishing pigs, a dose-response study. Report 723, Wageningen UR Livestock Research, Lelystad, The Netherlands. 2013;5.
25. Combs NR, Kornegay ET, Lindemann MD, Notter DR, Wilson JH, Mason JP. Calcium and phosphorus requirement of swine from weaning to market weight: II. Development of response curves for bone criteria and comparison of bending and shear bone testing. J Anim Sci. 1991;69:682–693.
26. ISO 17025. General requirements for the competence of testing and calibration laboratories. International Standards Organization, Geneva, Switzerland. 2005.
27. Van Soest PJ, Robertson JB, Lewis BA. Methods for dietary fiber, neutral detergent fiber, and nonstarch polysaccharides in relation to animal nutrition. J Dairy Sci. 1991;74:3583–3597.
28. Windisch W, Kirchgessner M. Zinkexkretion und kinetik des zinkaustauschs im ganzkörper bei defizitärer und hoher zinkversorgung. 2. zum effekt einer unterschiedlichen zinkversorgung auf den quantitativen zinkumsatz im stoffwechsel adulter ratten [Zinc excretion and kinetics of zinc in the whole body with deficient and high zinc supply. 2. The effect of zinc supply on the quantitative zinc turnover in the metabolism of adult rats]. J Anim Physiol Anim Nutr. 1994;71:123–130.
29. Windisch W, Kirchgessner M. Distribution and exchange of zinc in different tissue fractions at deficient and excessive zinc supply. 3. Effect of different zinc supply on quantitative zinc exchange in the metabolism of adult rats. J Anim Physiol Anim Nutr. 1994; 71:131–139.
30. Windisch W, Kirchgessner M. Zur messung der homöostatischen anpassung des zinkstoffwechsels an eine defizitäre und hohe zinkversorgung nach alimentärer 65Zn-markierung 1. Mitteilung zum effekt einer unterschiedlichen zinkversorgung auf den quantitativen zinkumsatz im stoffwechsel adulter ratten [Measure the homeostatic adaptation of zinc metabolism during a deficient and high zinc supply after alimentary 65Zn labeling 1. Information on the effect of zinc supply on the quantitative zinc turnover in the metabolism of adult rats]. J Anim Physiol Anim Nutr. 1994;71:98–107.
31. Windisch W, Kirchgessner M. Zinkverteilung und zinkaustausch im gewebe 65 Zn markierter ratten [Zinc distribution and zinc exchange in the tissue of 65 Zn labeled rats]. J Anim Physiol Anim Nutr. 1995;74:113–122.
32. Weigand E, Kirchgessner M. Total true efficiency of zinc utilization: determination and homeostatic dependence upon the zinc supply status in young rats. J Nutr. 1980;110:469–480.
33. Windisch W, Kirchgessner M. Anpassung des zinkstoffwechsels und des zinkaustauschs im ganzkörper 65Zn-markierter ratten an eine variierende zinkaufnahme. 1. Mitteilung. zum quantitativen zinkumsatz im stoffwechsel adulter ratten bei physiologisch adäquater zinkversorgung [Adjustment of zinc metabolism and zinc exchange in the whole body of 65Zn-labeled rats during a varying zinc uptake. 1. The quantitative zinc turnover in the metabolism of adult rats with a physiologically adequate zinc supply]. J Anim Physiol Anim Nutr. 1995;74:101–112.
34. Martinez MM, Link JE, Hill GM. Dietary pharmacological or excess zinc and phytase effects on tissue mineral concentrations, metallothionein, and apparent mineral retention in the newly weaned pig. Biol Trace Elem Res. 2005;105:97–115.
35. Martin L, Lodemann U, Bondzio A, Gefeller EM, Vahjen W, Aschenbach JR, Zentek J, Pieper R. A high amount of dietary zinc changes the expression of zinc transporters and metallothionein in jejunal epithelial cells in vitro and in vivo but does not prevent zinc accumulation in jejunal tissue of piglets. J Nutr. 2013;143:1205–1210.
36. Jollif JS. Evaluating dietary macro- and micromineral sources, levels, and their environmental impact in the porcine species. PhD Dissertation. The Ohio State University, Columbus, Ohio; 2011.
37. Kalinowski J, Chavez ER. Tissue composition and trace mineral content of the dam and litter under low dietary zinc intake during gestation and lacation of first-litter gilts. J Trace Elem Elect H. 1991;5:35–46.
38. Prasad AS, Oberleas D, Wolf P, Horwitz JP, Miller ER, Luecke RW. Changes in trace elements and enzyme activities in tissues of zinc-deficient pigs. Am J Clin Nutr. 1969;22:628–637.
39. Revy PS, Jondreville C, Dourmad JY, Nys Y. Bioavailability of two sources of zinc in weanling pigs. Anim Res. 2002;51:315–326.
40. Revy PS, Jondreville C, Dourmad JY, Nys Y. Effect of zinc supplemented as either an organic or an inorganic source and of microbial phytase on zinc and other minerals utilisation by weanling pigs. Anim Feed Sci Tech. 2004;116:93–112.
41. Revy PS, Jondreville C, Dourmad JY, Nys Y. Assessment of dietary zinc requirement of weaned piglets fed diets or without microbial phytase. J Anim Physiol Anim Nutr. 2006;90:50–59.
42. Schlegel P, Nys Y, Jondreville C. Zinc availability and digestive zinc solubility in piglets and broilers fed diets varying in their phytate contents, phytase activity and supplemented zinc source. Animal. 2010;4:200–209.
43. Jongbloed AW, van Diepen JTM, Binnendijk GP, Bikker P, Vereecken M, Bierman K. Efficacy of Optiphos™ phytase on mineral digestibility in diets for breeding sows: effect during pregnancy and lactation. J Livest Sci. 2013;4:7–16.
44. Neathery MW, Miller WP, Blackmon DM, Gentry RP, Jones JB. Absorption and tissue zinc content in lactating dairy cows as affected by low dietary zinc. J Anim Sci. 1973;37:848–852.
45. Sunder GS, Panda AK, Gopinath NCS, Rama Rao SV, Raju MVLN, Reddy MR, Kumar CV. Effects of higher levels of zinc supplementation on performance, mineral availability, and immune competence in broiler chickens. J Appl Poultry Res. 2008;17:79–86.
46. Girard CL, Robert S, Matte JJ, Farmer C, Martineau GP. Serum concentrations of micronutrients, packed cell volume, and blood hemogloblin during the first two gestations and lactations of sows. Can J Vet Res. 1996;60:179–185.
47. Gürdoğan F, Yildiz A, Balikci E. Investigation of serum Cu, Zn, Fe and Se concentrations during pregnancy (60, 100, and 150 days) and after parturition (45 days) in single and twin pregnant sheep. Turkish J Vet Anim Sci. 2006;30:61–64.
48. Fagari-Nobijari H, Amanloua H, Dehghan-Banadakyb M. Effects of zinc supplementation on growth performance, blood metabolites and lameness in young Holstein bulls. J Appl Anim Res. 2012;40:222–228.
49. Dermauw V, Dierenfeld E, Du Laing G, Buyse J, Brochier B, Van Gucht S, Duchateau L, Janssens GPJ. Impact of a trace element supplementation programme on health and performance of cross-breed (Bos indicus × Bos taurus) dairy cattle under tropical farming conditions: a double-blinded randomized field trial. J Anim Physiol Anim Nutr. 2015;99:531–541.
50. Bondzio A, Pieper R, Gabler C, Weise C, Schulze P, Zentek J, Einspanier R. Feeding low or pharmacological concentrations of zinc oxide changes the hepatic proteome profiles in weaned piglets. PloS One. 2013;8(11):e81202.
51. Pieper R, Martin L, Schunter N, Tudela CV, Weise C, Klopfleisch R, Zentek J, Einspanier R, Bondzio A. Impact of high dietary zinc on zinc accumulation, enzyme activity and proteomic profiles in the pancreas of piglets. J Trace Elem Med Biol. 2015;30:30–36.