Several naturally occurring exogenous compounds can occupy and stimulate estrogen receptors located in reproductive and other organs, thereby mimicking the action of endogenous estrogen. Pigs have two main estrogen receptors (ERα and ERβ), both are considered highly susceptible to exogenous mimics. Clinical outbreaks of hyperestrogenism due to oral intake of the heat-stable zearalenone mycotoxin derived from Fusarium contamination of cereals, particularly corn, are well documented in pigs and other mammals.1,2
The occurrence of hyperestrogenism due to oral intake of estrogenic isoflavonoids, such as genistein, daidzin, and daidzein is also well documented in rodents.3,4 but less so in pigs. Isoflavonoids particularly occur as phytoestrogenic components of soybean cultivars (Glycine max), and are much more abundant in its growing leaves and roots.5 Clinical outbreaks of hyperestrogenism have occurred in rodents unintentionally fed commercial diets containing soybean ingredient material with high levels of isoflavonoids.4 Analysis of these suspect diets indicated that clinical signs could occur with dietary levels of isoflavones in the order of 1000 to 24,000 µg/kg of feed.4 Dietary intake of soybean phytoestrogens has also been implicated in a range of human reproductive organ effects, such as infertility due to disruption of estrus and increased breast tissue density.6 Previous studies in pigs have been largely limited to in vitro and intentional challenge exposure studies.7,8 Testing of pig feed components for phytoestrogens has been minimal due to the limited availability and expensive nature of the high-performance liquid chromatography (HPLC) analysis required.
In this case study, we describe the clinical hyperestrogenic signs associated with the dietary intake of phytoestrogen isoflavonoids in 2 breeding herds.
Case description
Case farms and breeding herds
Breeding farms A and B under a single management group were located in the subtropical dry climate zone of mid Queensland, Australia. The breeding herd genetics for both farms were of a single source of synthetic Large White-Landrace crossbred pigs, with semen for artificial insemination supplied from a commercial boar stud. No new genetics were introduced to the farms between 2017 and 2019. Over this time, farms A and B had a mean population of 3550 and 1050 breeding age females, respectively, with weaned pigs taken to separate nursery and grower facilities. Female grower pigs were selected for re-entry to both breeding herds at 22 weeks of age based on leg conformation, teat conformation, and vulva characteristics. Gilt preparation consisted of physical boar exposure after 24 weeks of age. Gilts entered the estrus detection and mating program at 27 weeks of age, with the first mating upon second estrus at 30 to 36 weeks of age. Both breeding herds had been free of clinical signs or other evidence associated with parvovirus, classical swine fever virus, porcine reproductive and respiratory syndrome virus, and pathogenic porcine circovirus as monitored by ongoing necropsy, specific serology, and immunohistochemistry studies for 10 years preceding and throughout the case study period (2017-2019).
Monitoring of breeding pig performance
Breeding procedures on both farms were under the same management, and of industry standard to the same audited operating procedures. Staff retraining to all breeding procedures occurred under veterinary supervision 4 times each year. Breeding females were monitored for estrus by reaction to boar and back pressure test. Mating procedures consisted of artificial insemination with physical presence of a boar and placement of a back-brace boar simulator device. Insemination on both farms consisted of two 80 mL doses (24 hours apart) of Large White or Landrace semen from the same group of nucleus herd boars. Pregnancy confirmation was conducted via ultrasound device at 28 and 56 days post mating.
After farrowing, weaning occurred 22 to 28 days later. Two days after weaning, physical boar exposure was provided to sows until estrus and mating. A weaning-to-service interval of 7 days or less was considered normal. After 7 days, any unmated sows were placed into a separate “stales” pen, and further physical boar exposure provided.
Calculations were conducted weekly (June 2017 to June 2019) to determine incidence of estrus, conception and pregnancy rates (positive pregnancy at 28 and 56 days post mating, respectively), farrowing rates, wean-to-service intervals, litters produced per mated female per year, nonproductive days (breeding female days without pregnancy or lactation), number of piglets born alive, and piglets weaned per sow per year. Any clinical signs in the breeding pigs and offspring were recorded.
Diets and laboratory analyses
Farms A and B were each supplied separately by two unconnected feed mills (A1 and B1, 200 km apart), which both formulated pig stage-specific compound feeds. Each formulated diet consisted of proportionate wheat-barley-sorghum cereals with soybean or canola protein sources and other standard feed additives. Pigs were fed via dedicated silos, in-line augers, and pen hoppers. Any in-feed antibiotic medications were supplied by veterinary prescription and included in diets destined solely for the target group of pigs. Commercial mycotoxin adsorptive products (clay-silicate) were added to every diet at the dosages recommended by the manufacturers.
On two occasions in 2018 (February and May), 9 scoops of feed were taken from various pen hoppers and then homogenized for analysis, and this occurred on each farm. Analysis of these compound feeds was performed for known isoflavonoids by standard HPLC analysis coupled with mass spectrometry (MS).9 In brief, 5 g of each feed sample was extracted by acetonitrile/water/acetic acid for 90 minutes, diluted, then screened and quantified in selected reaction monitoring mode within the HPLC/MS (Agilent biosystems, IFA-Tulln, University of Natural Resources and Life Sciences, Vienna, Austria). Two transitions leading to 4 identification points were established for each analyte, with calibrations performed via serial dilutions of a multi-analyte stock solution.
For mycotoxin testing, the feeds were also sampled in the same manner every 2 months (n = 10). Analysis of these compound feeds was performed for 23 known mycotoxins by a commercial liquid-liquid analysis coupled with mass spectrometry (Spectrum 380; Biomin).
Case investigations
Pregnant breeding pigs were fed a stage-specific diet for gestation (dry sow diet) then switched to a lactation diet starting at 10 days prior to farrowing, then reverting to the dry sow diet after the next estrus and remating. At farm A only, an additional diet (also from the feed mill A1) was provided for the females during the weaning-to-service period. Formulations for these diets varied slightly over the 2 years depending on ingredient availability. Feed mills A1 and B1 respectively formulated 500 and 100 metric tons of pig feed weekly, and therefore formulated 11 and 8 diets, respectively, over the study period. The median and range of ingredients used in these diet formulations are listed in Table 1. The cereal, oil, and additive diet components were generally similar, however, soybean meal and mill run (feed mill remainder) content of lactation and wean-to-service diets were consistently higher in farm A diets, whereas canola meal and chickpeas were used more in farm B diets (Table 1). Dietary ingredients for feed mills A1 and B1 were supplied from different local and global sources. The soybean meal used in feed mill A1 was supplied by a major global feed company and sourced via container from Argentina. The imported soybean meal had been emptied and held in a single bulk silo at a port distribution center. This bulk silo supplied soybean meal to feed mill A1 every 2 weeks.
Diet ingredient, kg/metric ton* | Feed mill A1† | Feed mill B1† | |||
---|---|---|---|---|---|
Gestation | Lactation‡ | Wean-to-Service | Gestation | Lactation‡ | |
Barley | 350 (200-500) | 200 (100-220) | 200 (100-370) | 450 (290-500) | 200 (200-200) |
Sorghum | 300 (0-430) | 100 (0-100) | 200 (0-400) | 190 (100-460) | 100 (80-150) |
Wheat | 0 (0-140) | 320 (270-450) | 250 (0-430) | 100 (0-230) | 450 (300-490) |
Mill run | 200 (150-200) | 120 (100-160) | 120 (100-200) | 0 | 0 |
Chickpea | 0 | 0 | 0 | 100 (100-100) | 50 (0-100) |
Vegetable oils | 25 (10-27) | 25 (15-37) | 16 (10-28) | 25 (0-50) | 15 (7-18) |
Canola meal | 60 (50-120) | 50 (0-120) | 60 (40-90) | 70 (0-120) | 75 (60-100) |
Soybean meal | 0 | 120 (60-130) | 85 (55-90) | 0 | 30 (0-40) |
Meat meal | 25 (15-45) | 25 (15-50) | 30 (20-60) | 10 (10-15) | 25 (15-50) |
Alfalfa crumble | 10 (0-12) | 10 (6-12) | 10 (6-12) | 25 (25-25) | 15 (12-18) |
* Portions of salt, mycotoxin adsorptive product, synthetic amino acids, and mineral premix were added to all diets in standard amounts, each less than 5 kg/metric ton.
† Feed mill A1 supplied farm A (affected) with 11 formulations between 2017 and 2019. Feed mill B1 supplied farm B (unaffected) with 8 formulations over the same time.
‡ Lactation diets were analyzed for mycotoxin and phytoestrogen content.
For farms A and B, conception rates were 96.4% and 98.6%, pregnancy rates were 95.0% and 98.4%, and farrowing rates were 86.6% and 91.5%, respectively (Figure 1). The litters farrowed per mated female per year were 2.32 and 2.43 and pigs weaned per sow per year were 23.7 and 24.7 on farms A and B, respectively (Figure 2). The wean-to-service interval was 6.8 and 6.4 days and nonproductive days were 40.8 and 30.4 on farms A and B, respectively (Figure 3). The number of piglets born alive per litter (11.7 and 11.6) and the number of piglets weaned per litter (10.2 and 10.1) were similar in farms A and B. We therefore noted a consistently better breeding performance in pigs on farm B (considered unaffected).
Clinical signs were only evident in 5% to 10% of breeding females on farm A during the approximate 120 farrowing events per week. Typical clinical signs were vulval tumefaction (red, swollen, “glassy” vulvas; Figure 4) and failure of proper mammary development noted upon entry to the farrowing area. This failure of mammary development generally resolved within 4 days after farrowing. Approximately 5% of sows on farm A showed estrus behaviour at 18 to 24 days after farrowing. These behaviours included chomping and restlessness, pricked ears, and clear vaginal discharge. The wean-to-service interval was noticeably delayed on farm A (Figure 3) with 7% to 14% of sows showing estrus behaviour 15 to 20 days post weaning. Intermittently, vulva reddening and swelling was observed in female piglets (born on farm A in May 2018) at birth until 5 days of age. None of these findings were noted on farm B; the pigs’ genetic origin and farm management procedures, including breeding, data collection, operating, staff training, and auditing procedures, were considered identical.
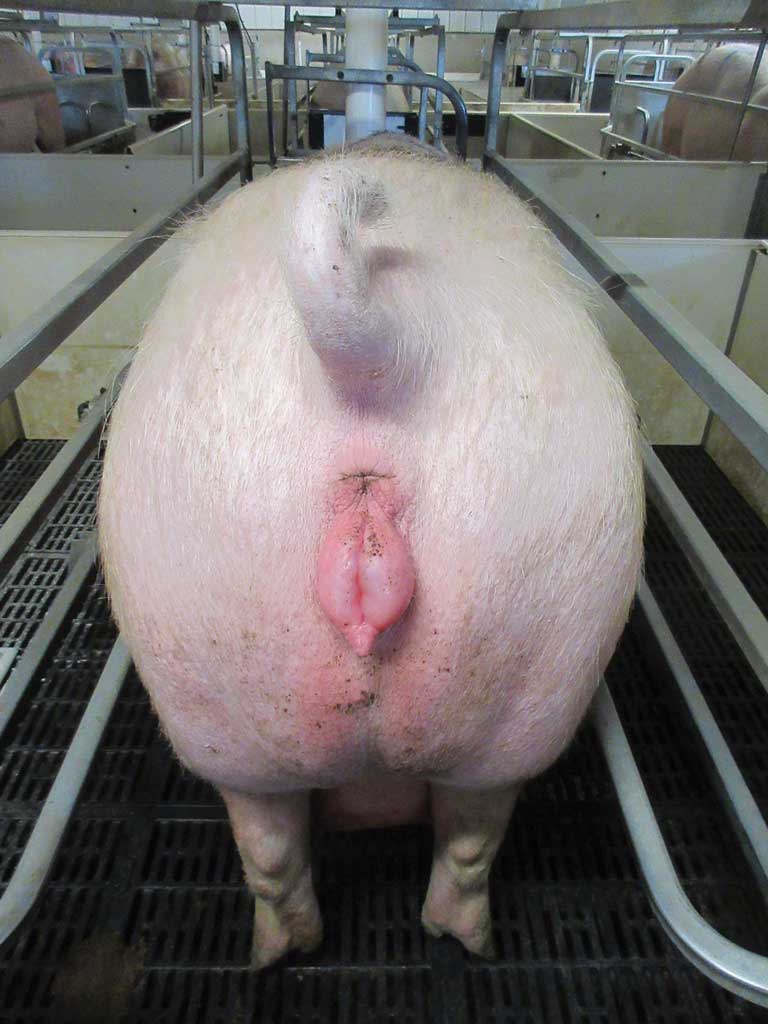
Phytoestrogenic typing
Analysis of compound feeds derived from feed mill A1 and consumed by females on farm A before, during, and after lactation showed high levels (up to 55,977 µg/kg) of several isoflavonoids, including genistin, glycitin, and daidzin, (Table 2). Scrutiny of dietary ingredients in the affected and unaffected farms (Table 1) indicated that the dietary presence of these isoflavonoids was associated with one source of soybean meal component at feed mill A1, derived from a batch imported from Argentina by a major global feed commodity group and held in a bulk silo. This soybean batch was used throughout the testing period in 2018. Repeated testing (10 occasions) of feed on farms A and B indicated 23 known mycotoxins, including zearalenone, remained below detectable levels or within reference ranges for no effect (Table 2). A change of soybean materials in feed mill A1 was instituted at the end of the study period (September 2019) and the clinical syndrome then dissipated.
Farm A Lactation diet | Farm B Lactation diet | |
---|---|---|
Mycotoxin,*µg/kg | ||
T-2 toxin | 1.86 | 1.90 |
Fumonisin B-4 | 2.44 | 2.50 |
Zearalenone | ND | ND |
Phytoestrogen, µg/kg | ||
Daidzein | 1045 | ND |
Daidzin | 53,658 | ND |
Genistein | 1733 | ND |
Genistin | 55,977 | ND |
Glycitein | 328 | ND |
Glycitin | 12,713 | ND |
* Twenty other mycotoxins were analyzed but below the limit of detection including aflatoxins B1, B2, G1, and G2, fumonisin B1, B2, and B3, ochratoxin, nivalenol, deoxynivalenol, 3-acetyl deoxynivalenol, 15-acetyl deoxynivalenol, HT-2 toxin, fusarenon X, neosolaniol, deacetoxyscirpenol, enniatin A1, B, and B1, and ergot alkaloid group.
ND = below the limit of detection.
Discussion
In this case study, we identified the presence of clinical hyperestrogenism in a proportion of breeding females on an affected farm with the presence of isoflavonoids in specific lactation and wean-to-service diets. These isoflavonoids were apparently derived from one source of soybean meal and were not present to the same extent in the farm B diets. Isoflavonoids are not known to be present in cereals or major dietary components of pig diets, other than soybean meal. The clinical signs noted in affected breeding animals included vulval tumefaction, mammary gland dysfunction, and various signs of infertility, such as more nonproductive days, presumably due to estrogenic effects on the ovary and uterus. These signs closely mimicked those seen in hyperestrogenism syndrome due to zearalenone toxicosis,1 but analysis of feed for this and other mycotoxins was consistently negative and constant use of commercial mycotoxin binders in the farm diets did not alleviate the syndrome.
Previous studies of phytoestrogens in pigs have been largely limited to in vitro and intentional challenge exposure studies.7,8 This case study documents the occurrence of hyperestrogenism associated with an oral intake of phytoestrogenic isoflavonoids in commercial farm pigs. The limitations of the case study include that the side-by-side comparison of breeding parameters and feed investigations were only possible on this farm system, rather than all regional pig farms experiencing similar clinical signs. The activity of the isoflavonoids detected in the diets, particularly genistin and daidzin, are well-documented as causes of hyperestrogenism when present in the diet of laboratory animals and other mammals.3,4 In contrast to mycotoxin testing, the testing of pig feeds for phytoestrogens by HPLC has limited availability and is not widely conducted. Phytoestrogenic isoflavonoids and mycotoxins are both potent stimulators of ERα and ERβ. Because no myco-estrogens were detected in the pig feeds in this case study, we therefore considered the isoflavonoids to be involved in the hyperestrogenism syndrome in these sows.
Isoflavonoids occur as phytoestrogenic components of soybean cultivars and are particularly abundant in its growing leaves and roots.5 The commercial preparation of batches of soybean meal can incorporate approximately 20% of so-called soybean trash, which usually includes leaf and other non-bean components.10 It is possible that the particular batch of soybean meal used during this case study contained a noticeable quantity of such soybean trash. The aggregation of soybean batches from a variety of in-country sources into this commercial soybean meal, did not allow more specific source identification. Corn and soybean ingredients are used to a lesser extent in feed mill preparation of pig diets in Europe, Asia, and Oceania compared to American diets due to transportation costs. The percentage of soybean meal within suspect diets at feed mill A1 was below standard inclusion rates for some American pig diets. Unlike mycotoxin testing, the testing of soybean meal and other ingredients for phytoestrogens is not a routine practice. Unlike established mycotoxin inhibitor usage, there is limited availability of products that inhibit dietary phytoestrogens. It is therefore suggested that future clinical cases of hyperestrogenism in pigs be investigated for these two possible sources of endocrine disruptors.
Clinical outbreaks of hyperestrogenism have also occurred in housed rodents unintentionally fed commercial diets containing soybeans with high levels of isoflavonoids.4 Measurement of the isoflavonoid levels in these commercial rodent diets derived from suspect soybean ingredient materials indicated that clinical signs could occur with dietary levels of isoflavonoids in the order of 1000 to 24,000 µg/kg of feed,4 which were similar to or less than the levels of the isoflavonoids noted in the suspect pig diets in this case study.
Isoflavonoids are known to be absorbed and distributed quickly to the reproductive organs after oral ingestion.3,7 In this case study, we therefore associated the intake of suspect soybean meal in lactation diets and subsequent postweaning sow diets with onset of hyperestrogenism signs of infertility, particularly noticeable in the wean-to-service phase. The exact dose of isoflavonoids required to precipitate hyperestrogenism in sows in a farm setting is not clear from previous studies.7 A calculation of isoflavonoids present in the analyzed pig diets and a normal 200 kg sow average feed intake of approximately 6 kg/day would tend to indicate that the clinical signs noted in this case study could occur with approximately 1 kg of soybean meal content containing approximately 0.12 kg of isoflavonoids (Table 2) per day, that is 0.6 mg of isoflavonoids per kg of bodyweight. It is reasonable to expect that the severity of signs would be dose dependent.
Implications
Under the conditions of this study:
- Soybean-derived isoflavonoids were associated with hyperestrogenism in sows.
- Clinical signs were vulval tumefaction, mammary gland dysfunction, and infertility.
Acknowledgments
We thank the Biomin Inc staff for assistance in organizing the excellent laboratory analyses. We also thank the diligent farm managers for the original pig performance data collection.
Conflict of interest
None reported.
Disclaimer
Scientific manuscripts published in the Journal of Swine Health and Production are peer reviewed. However, information on medications, feed, and management techniques may be specific to the research or commercial situation presented in the manuscript. It is the responsibility of the reader to use information responsibly and in accordance with the rules and regulations governing research or the practice of veterinary medicine in their country or region.
References
1. Aucock HW, Marasas WF, Meyer CJ, Chalmers P. Field outbreaks of hyperoestrogenism (vulvo-vaginitis) in pigs consuming maize infected by Fusarium graminearum and contaminated with zearalenone. J S Afr Vet Assoc. 1980;51:163-166.
2. Fink-Gremmels J, Malekinejad H. Clinical effects and biochemical mechanisms associated with exposure to the mycoestrogen zearalenone. Anim Feed Sci Technol. 2007;137:326-341.
3. Franke AA, Halm BM, Kakazu K, Li X, Custer LJ. Phytoestrogenic isoflavonoids in epidemiologic and clinical research. Drug Test Anal. 2009;1:14-21.
4. Brown NM, Setchell KDR. Animal models impacted by phytoestrogens in commercial chow: implications for pathways influenced by hormones. Lab Invest. 2001;81:735-747.
5. Morgan HE, Dillaway D, Edwards TM. Estrogenicity of soybeans (Glycine max) varies by plant organ and developmental stage. Endocr Disruptors. 2014;2(1):e28490. doi:10.4161/endo.28490
6. Patisaul HB, Jefferson W. The pros and cons of phytoestrogens. Front Neuroendocrinol. 2010;31:400-419.
7. Ford JA Jr, Clark SG, Walters EM, Wheeler MB, Hurley WL. Estrogenic effects of genistein on reproductive tissues of ovariectomized gilts. J Anim Sci. 2006;84:834-842.
*8. Brookshire C, Necaise K, Moulton K, Christiansen D, Crenshaw M, Scanes C, Ryan P. Evaluation of thermal gradients of the external genitalia of neonatal pigs following oral exposure to the soy-derived phytoestrogen genistein and estradiol. Proc AASV Annual Meeting. Dallas, TX. 2009:307-308.
9. Vishwanath V, Sulyok M, Labuda R, Bicker W, Krska R. Simultaneous determination of 186 fungal and bacterial metabolites in indoor matrices by liquid chromatography/tandem mass spectrometry. Anal Bioanal Chem. 2009;395:1355-1372.
10. Thakur M, Hurburgh CM. Quality of US soybean meal compared to the quality of soybean meal from other origins. J Amer Oil Chem Soc. 2007; 84: 835–843.
* Non-refereed reference.